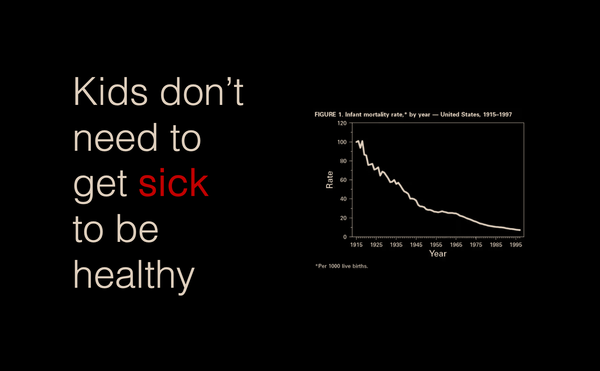
immunology
Kids don't need to get sick to be healthy
The 'Make America Healthy Again' movement is tapping into the commonly held belief that children in our modern era are less healthy than they used to be. Fueling this sentiment is the 'Hygiene Hypothesis' – an idea postulated in the 1980's that modern, overly sterile